High altitude balloons have recently come under a lot of criticism. In February, the US military fired down two balloons: a spy balloon that might have been operated by the Chinese government and a “unknown aerial phenomenon” that was later shown to be most likely a hobby balloon.
Early in May, when people saw another large balloon in the southern hemisphere, there was concern that it might be a new espionage device. Instead, it represents the astronomy of the future: deep-sky telescopes carried by balloons that never have to leave the stratosphere.
William Jones, a physics professor at Princeton University and the leader of NASA’s Super Pressure Balloon Imaging Telescope (SuperBIT) project, declares, “We’re searching for, not down.” The nearly 10-foot-tall telescope, which was launched from Wnaka, New Zealand, on April 15, has already completed four complete rotations of the southern hemisphere on a balloon the size of a football stadium constructed of polyethylene film. Additionally, its three onboard digital cameras captured breathtaking images of the Antennae and Tarantula Nebula galaxies that matched those taken by the Hubble Space Telescope. The results from SuperBIT could help scientists unlock one of the greatest mysteries of deep space: the nature of dark matter, an element that is theoretically invisible but whose gravitational effects on visible objects provide the only evidence of its existence.
[Related: $130,000 could buy you a Michelin-star meal with a view of the stars]
Next-generation observatories, like the James Webb Space Telescope, can be used to study dark matter by relying on their large mirrors and locations away from Earth’s harsh environment to obtain stunning views of exceedingly far-off astronomical objects. It costs a lot of money to build an area telescope and launch it with a powerful rocket. For instance, it cost about $1.5 billion to launch Hubble into orbit and over $10 billion to launch JWST to Lagrange Point 2.
Due to the relative affordability of balloons compared to rockets and the reduced entrance barrier for qualified people to develop the system, SuperBIT’s launch cost under $5 million.
“Trainees are in charge of everything. That’s what makes jobs like these so dynamic and capable of doing a lot with little funding, says Jones, referring to the SuperBIT collaboration involving Princeton, the University of Toronto in Canada, and the University of Durham in the United Kingdom. Only college students have the luxury of being able to give the assignment their full-time attention because we don’t have any expert engineers or specialists working on it full-time.
SuperBIT was not the first telescope to be launched with a balloon; that distinction belongs to Princeton’s Stratoscope I, which was built in 1957. One of the few brand-new observatories made possible by NASA’s 20 years of research into so-called extremely pressure balloons is SuperBIT. The testing flights that began in 2015 and the ground-breaking launch of SuperBIT were the final results of that endeavour.
Traditional balloons contain a lifting gas that expands when the sun warms it and as height changes the air pressure. This changes the volume of the envelope, which affects the buoyancy of the balloon and makes it challenging to maintain a constant elevation over time.
Superpressure balloons maintain the pressure inside a primary envelope of the lifting gas, often helium, so that volume and buoyancy remain constant during the day and night. A smaller balloon, called a ballonet, is then used by the balloon as ballast inside or beneath the main envelope, filling or emptying the pocket with compressed air to change height and effectively control the ship.
With a 3,500-pound payload of medical equipment, SuperBIT’s extremely pressurised balloon can maintain an altitude of 108,000 feet (higher than 99.2 percent of Earth’s environment). The purpose of the SuperBIT telescope is not to see deeper or wider areas of deep space or to find exoplanets, unlike JWST and other goals. Instead, it is looking for signs of a more widespread and enigmatic entity.
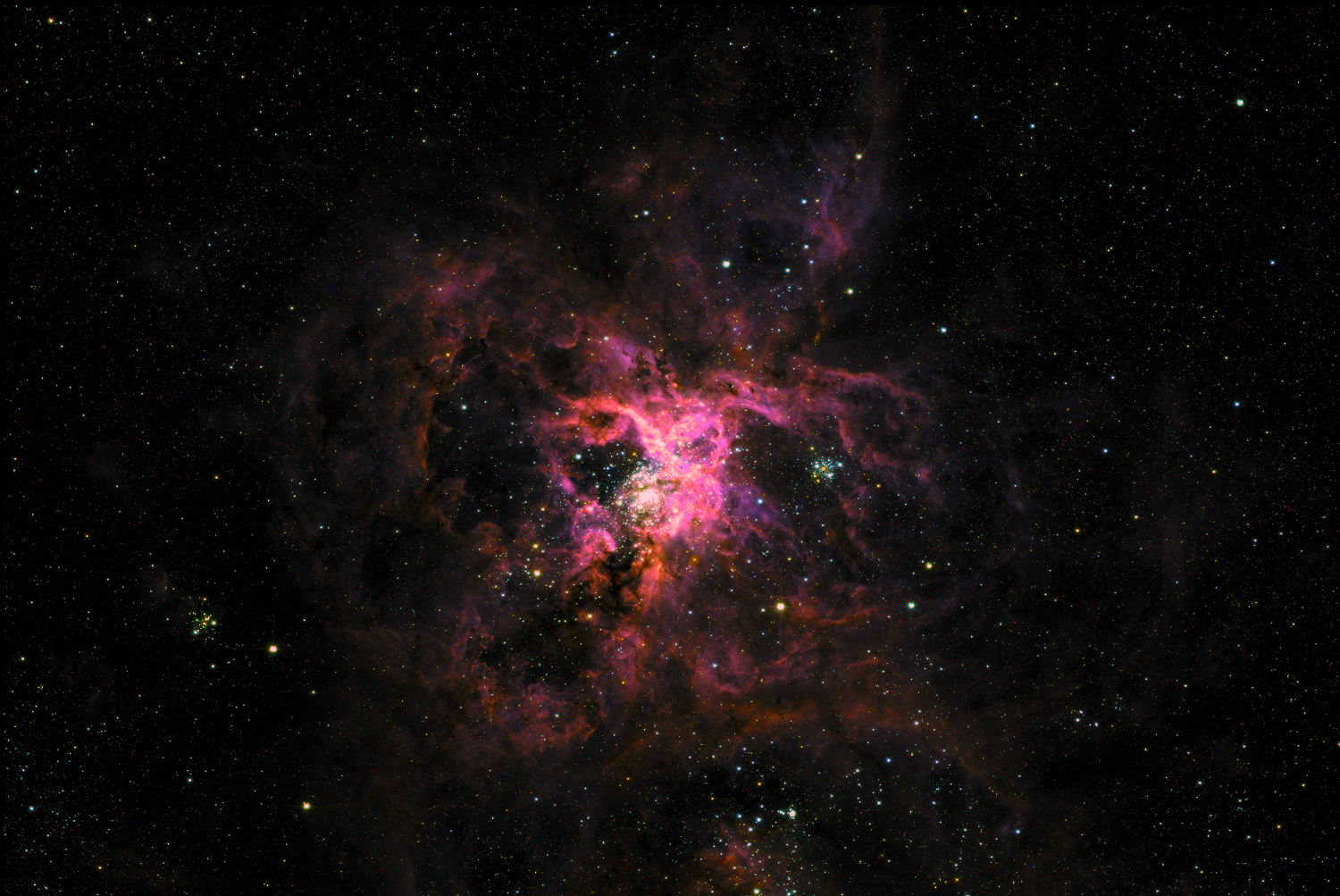
According to Jones, “Dark matter is not made from any of the components or particles that we can identify through routine observations.” That being said, there is a lot of stuff nearby: stuff may make up around 27% of deep space. According to Jones, “We understand this through the gravitational impact that it has on the normal matter—stars and gas and so on—that we can see, which constitutes around 5% of deep space.
Researchers estimate that dark energy, another primarily weird component that should not be confused with dark matter, makes up 67 percent of the remaining universes. Dark energy may be responsible for the universe’s expanding more quickly than dark matter, which may help pull galaxies together and shape how they populate their universes.
By observing star clusters so massive that their gravity bends the light passing by them from more distant objects—a technique known as gravitational lensing—scientists probe extreme forces where dark matter may exist and determine its existence. Using this method, astronomers can use galaxies as a kind of magnifying lens to observe more distant objects than they typically could (a feat that JWST excels at). Additionally, it can reveal the mass of the star clusters that make up the “lens,” as well as the amount of dark matter that surrounds them.
“We’re trying to figure out what dark matter is,” says Richard Massey, a member of the SuperBIT research team and a physics instructor at Durham University. “After figuring out how much there is and where it is, we’ve got to figure out what it is.” We accomplish this by examining a few special places in deep space where clumps of dark matter are observed to be colliding with one another.
These places include the two large Antennae galaxies, which are still colliding roughly 60 million light-years from Earth. Hubble has been used by Massey and others to study the Antennae galaxies, but Massey complains that it “offers it a field of vision too little to see the titanic crashes of dark matter.” So, SuperBIT development was required.
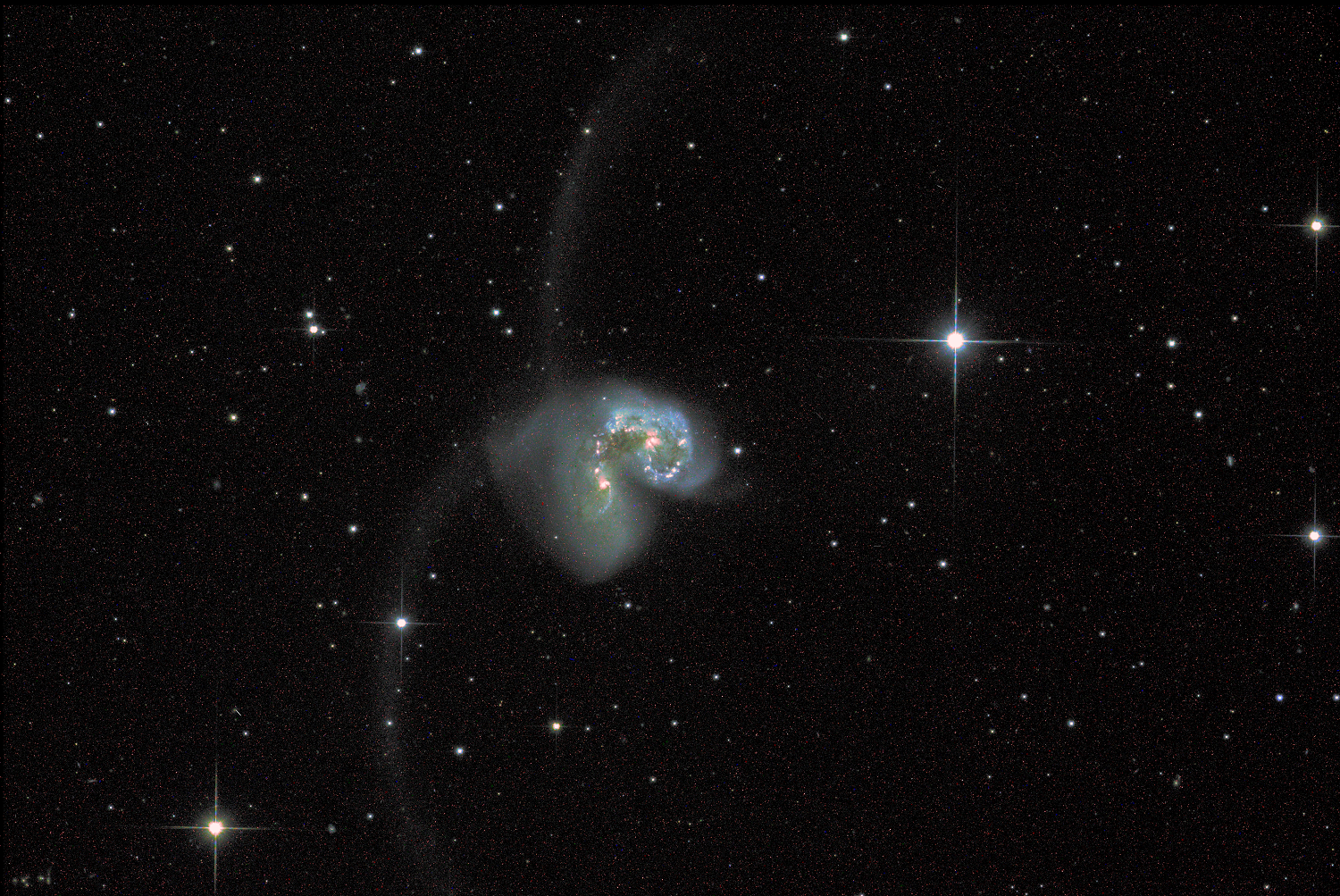
SuperBIT observes light with wavelengths between 300 and 1,000 nanometers, similar to Hubble. SuperBIT can picture bigger regions of the sky at once since its field of view is half a degree, compared to Hubble’s maximum field of view, which is less than a tenth of a degree. That’s true even though its mirror is smaller than Hubble’s (half a metre against 1.5 metres in size).
Compared to local telescopes, SuperBIT offers additional advantage. The SuperBIT team was able to use even more advanced cam sensing units than those on existing area telescopes since it took less time from development to implementation and didn’t need complicated equipment to protect it from radiation, extremely high temperatures, and space particles. Jones claims that SuperBIT consists of a 60-megapixel sensing unit as opposed to Hubble’s Wide Field Camera 3’s collection of 8-megapixel sensing units. The balloon-carried telescope is also designed to drift back to earth on a parachute after each flight, allowing scientists to regularly update the technology from the ground.
For the next 100 days, “we are interacting with SuperBIT live, 24 hours a day,” says Massey. It recently finished its fourth tour around the world, taking in the southern lights, turbulence above the Andes, and quiet cold above the centre of the Pacific Ocean. According to Jones, the gang plans to recover the system sometime in late August, probably in southern Argentina.
[Related on PopSci+: Alien-looking balloons could be the next firefighting tool]
SuperBIT might only be the beginning. A Gigapixel class Balloon Imaging Telescope (GigaBIT), which will have a mirror as big as Hubble’s, is currently being funded by NASA. GigaBIT would not only be “much more effective than anything likely to be taken into space in the near term,” according to Jones, but it is also anticipated to be less expensive than any space telescope observing the same spectrum of light.
It is too soon to say whether SuperBIT will uncover the precise nature of dark matter. The college student will need to read the task’s findings after a few flights.
What will the [data] tell us, exactly? Who comprehends! That’s the fun of it—as well as the dishonest trick,” says Massey. We still have no idea of what the two most common types of things in deep space are or how they behave, despite 2,000 years of science.